I hope many of you have read my Climate Change parts 1, 2 & 3 Blogs. This week I’m going to revisit and update those blogs with an emphasis on how to solve the worldwide global warming problem in ways that benefit humanity. Unfortunately, our newly elected administration wants to punish carbon emitters with taxes (which raises energy costs for you and me), instead of rewarding carbon-reduction solvers with research contracts (which can fix the problem while reducing energy costs for you and me). I have hopes that their philosophy might shift when they get into office, but I’m not going to hold my breath.
The global carbon emitters are shown in figure 1 below. The biggest emitter is base load and variable electricity generation, and real progress has been made there by embracing renewable energy sources (Photovoltaic and Windmills) and replacing coalfired plants with cleaner and more efficient natural gas turbines. Final elimination will require nuclear powerplants.

Figure 1 Global Fossil Fuel and Industry Emissions
The next biggest carbon emitter is short and medium range transportation (cars & trucks). Real progress is made here also with electric cars and trucks starting to make real inroads in current and future sales predictions. There are still unresolved issues with having enough charging stations and the fact that the majority of our electricity is still generated using fossil fuels. In effect, California eliminating sales of fossil-fueled cars and trucks by 2035 will drive up the states demand for electricity by 30% to 40% in a few years, and most of those cars will be charged at night when the sun doesn’t shine.
As you can see in figure 1 roughly 3/4 of carbon emissions are directly or can be indirectly generated by electricity (electricity could be substituted for short-range transportation and fossil fuel heating), hence the key issue to be solved in global warming is what power source are we going to use to generate electricity when the sun isn’t shining, and the wind isn’t blowing? There are two possibilities, batteries and nuclear power, and the correct answer should probably be determined by economics, not politics.
Backup battery power for renewable sources is probably the simplest and most straightforward solution to intermittent loss of power. However, battery capital costs are very much a function of the duration of the power outage covered (see figure 2 below) so I will assume we can get by with 6-hour batteries without occasional electrical blackout from nightfall or no winds.

Figure 2 Cost Projections for backup Batteries (Utility Electrical System)
As you can see the experts are predicting very rapid reductions in battery costs over the next thirty years. This is probably driven by the assumed massive switch to electrical vehicles over the next few years. Please note that the baseline renewable power system must be oversized by roughly 30% if batteries are going to be continuously charged and used for an average of 6 hours out of 24. This assumes 10% losses during charging and 10% during discharging of the batteries. Unfortunately, the cost of the batteries plus the 30% size increase makes an all wind and solar system noncompetitive with a system combining wind and solar with natural gas.
In the United States right now the largest fuel source is natural gas, accounting for more than 44 percent of all electrical generation capacity. Coal is second, with a share of just under 21 percent. Nuclear, hydro, and wind together account for just over one-quarter of capacity, and Solar currently constitutes over three percent of all capacity. Over 348,000 MW of new generation capacity is under development in the United States. Wind and natural gas both account for 36 percent of the new generating capacity under construction or permitted, and solar accounts for most of the remaining capacity in these categories. Natural gas, solar, and wind projects account for 94 percent of all capacity under construction. Of the capacity slated to begin operating in 2020, 99 percent will be fueled by these three resources, with wind and solar accounting for more than 70 percent of new capacity. As you can see batteries are not in current plans because natural gas-fired combined-cycle powerplants are both cheap and efficient, plus function as variable power sources to add flexibility to the overall power grid.
My position is that the electric power utilities will gradually switch to all wind, solar, and natural gas as power sources, based on lower capital costs plus operating costs, and not budge from that position until a still lower cost alternative appears (probably a new small molten salt fission reactor or small fusion reactor). Once a new safer, cheaper nuclear powerplant is available, the natural gas plants will be phased out.
How about the other 15% of the carbon emissions in figure 1 we haven’t talked about yet? That includes 1% for Long-Haul Trucking, 2% for aviation, 3 % for shipping, 4% for cement production, and 5% for iron and steel production. For Long-Haul Trucking, Aviation, and shipping I recommend switching first to natural gas and then to hydrogen for the Diesel fuels currently in use. Eventually, hydrogen will win out because it is carbon free. For long haul trucking hydrogen fuel cells are already being proposed by every major truck manufacturer. For Aviation fuel cells are probably too heavy so hydrogen burning turbine engines will be the norm. For rail shipping I imagine the rail lines will be electrified to match most of the developed world, but fuel cells could win out in remote areas.
Cement production currently burns coal to heat the limestone and drive off the CO2. MIT has recently proposed an electrolysis technique that generates pure CO2 combined with H2 at the anode and pure O2 at the cathode. In the process limestone (CaCO3) is converted to Ca(OH)2 which can be used to make Portland Cement without any greenhouse emissions. The pure CO2 can be easily separated from the H2 and sequestered deep underground while the H2 and the O2 can be used for energy generation.
Iron and steel production currently burn coke to drive the oxygen out of iron ores, but an electrolysis-based method has been proposed by Professor Stuart Licht of George Washington University to extract metallic iron from iron ores without carbon emissions. The technique shown in figure 3 below relies on the finding that iron ores are soluble in molten carbonate at high temperatures. The oxygen in the dissolved iron ore is released at the anode while iron is plated on the cathode as shown. No carbon is released using this process.
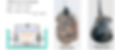
Figure 3 Electrolysis of dissolved Iron Ores
I have shown above how to most if not all of the carbon emissions over time but what have these solutions have to do with mining the moon?
That answer has to do with the implementation details of the technologies I have embraced. Windmills are obviously going to be a major player in future electrical power generation. In the US I estimate 600 billion kW-h of wind generated energy by 2050 according to reference: (https://www.eia.gov/todayinenergy/detail.php?id=38252). At a 25% availability rate this requires 2.74 Million kW of installed windmill powerplants. A one MWe wind turbine generator uses 1 mT of Neodymium in its magnetic coils so by 2050 we are going to use 2,740 mT of Neodymium for US windmills and roughly 1.1 Million mT for the rest of the planet (see global historical energy consumption in figure 4 below).

Figure 4 Global Primary Energy Consumption
Note that the annual world production of neodymium oxide stands at 7,000 tonnes, and the total reserves are estimated at about 8,000,000 tonnes. Neodymium oxide is 95% neodymium by weight, so we are going to have to increase our mining rate by over a factor of five to meet the global windmill requirement. Or we can find a new source off planet. Neodymium is a Rare Earth Element (REE) and is one of seventeen elements that are relatively hard to mine on Earth but readily available in a certain region on the moon’s near side., Note, that because of their geochemical properties, rare-earth elements are typically dispersed and not often found concentrated in rare-earth minerals, so economically exploitable ore deposits are less common. Other pertinent uses of REEs are typical electric automobiles. A Toyota Prius uses I kg of Neodymium in its traction motor and 15 kg of Lanthanum in its batteries. Yttrium, another REE, is found in high Temperature Superconductors (critical to fusion reactors)), television screen, and high-power glass lasers. I am not to cover all the REEs here, but they have multiple uses is a high technology society.
The other class of elements in abundance on the moon (and also the asteroids) are the Platinum Group Metals (PGMs). The six platinum-group metals are ruthenium, rhodium, palladium, osmium, indium, and platinum. They have similar physical and chemical properties and tend to occur together in the same mineral deposits. In this case they are found dissolved in nickel-iron fragments scattered across the lunar surface from asteroid impacts back in antiquity. PGMs are frequently found with gold and silver as contaminants. My University of Washington Senior Space Design class back in 2012 designed and analyzed a commercial venture to mine PGMs and REEs on the lunar surface and they concluded it was doable and very profitable (36% Return on Investment (ROI)).
In our carbon-free future PGMs are vital catalysts in the hydrogen fuel cells which give the highest efficiency and longest life. One reason fuel cells have not replace batteries is the high cost of platinum. Platinum from the moon should be cheaper given that platinum is very scarce in the Earth’s crust. If long-haul trucking and shipping convert to hydrogen fuel cells, they will need the additional PGMs found on the moon. Similarly, with the entire planet converting to electric cars and trucks we will also need the abundance of REEs found on the moon to build the motors and batteries required.
Finally, if we get to the point where a fusion reactor feasible and economically attractive, a low-radiation nuclear fuel is D-He3 and the only nearby source of He3 is the moon. It has been embedded in the lunar regolith by the solar wind and can be harvested economically with the right equipment (see my book).
Thanks for reading.
Dana Andrews
References
Cole, Wesley, and A. Will Frazier. 2020. Cost Projections for Utility-Scale Battery Storage: 2020 Update. Golden, CO: National Renewable Energy Laboratory. NREL/TP-6A20-75385.
https://www.nrel.gov/docs/fy20osti/75385.pdf
https://www.publicpower.org/system/files/documents/Americas-Electricity-Generation-Capacity-2020.pdf
https://phys.org/news/2010-08-carbon-dioxide-free-method-iron.html#:~:text=The%20process%20of%20producing%20iron%20free%20of%20carbon,temperatures%20is%20significantly%20more%20soluble%20than%20previously%20thought.