Space Exploration Part 2
- Dana G. Andrews
- Nov 3, 2019
- 13 min read
Updated: Nov 17, 2019
This is the second part of an Analysis of the current US Space Program.
In this part (Part 2) we show the best approach for putting permanent outposts as well as profitable mining bases on the moon. Next, we show what it is really going to take to put a permanent base on Mars.
Space Program Part 2–What is the best way to Return to the Moon, and then Colonize Mars?
There is a controversy about the method for to returning to the moon that almost none of you know about. NASA has ground ruled the mandatory use of their Gateway Station as a way station located in high lunar orbit (3000 by 70,000 km). The idea is that the Orion capsule would be boosted from Low Earth Orbit (LEO) by an expendable Centaur upper stage, then separate and rendezvous and dock with the Gateway Station using the Orion propulsion stage.The Orion would stay there while the astronauts transfer to the lunar lander based at the Gateway Station and depart for the lunar surface. Doctor Robert Zubrin, an old friend, has publicly stated that is a dumb idea and proposed to use a larger lander that can travel from Low Earth Orbit (LEO) directly to the lunar surface. His approach is certainly better than NASAs, but I did numerous studies for NASA during the 1990s and early 2000s examining the best way to develop the moon and I would propose a third way to return to the moon which is better than either of the other two. The comparison between the three approaches is detailed below.
Travelling from the Earth to the Moon -
To really understand the differences in the three approaches we need to understand the orbital mechanics of travelling from the Earth to the Moon. First, the moon orbits the Earth at a mean distance of 385,000 km in an orbit inclined 5.1 degrees to the ecliptic (plane of Earth’s orbit around the sun), but the moon equatorial plane is only inclined 1.5 degrees to the ecliptic. This is why crater bottoms at the poles never see sunshine. If we assume our spacecraft is launched into an Earth orbit inclined at 28.5 degrees to the Earth’s equator (launch point at Cape Canaveral, or a space station at 28.5 degrees) and we going to use direct Earth-to-Moon trajectories, then we are limited to two opportunities per lunar month (when the moon at arrival time crosses the 28.5-degree orbit plane). This shown in Figure 3 below in a rotating frame of reference.
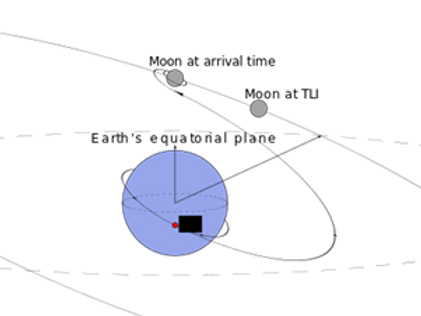
Within the constraint of direct Earth-to-Moon trajectories, there is a variety of transfer times and DVs to choose from. These are shown in Figure 4 below. In this table C3 is the characteristic energy of the transfer trajectory and is equivalent to the orbital velocity squared of the current trajectory at infinity. For an Earth orbit C3 is negative, for a parabolic trajectory C3 = 0, and for a hyperbolic (escape) trajectory, C3 is positive. C3 is an easiest way to characterize the total energy of a particular trajectory and will be an important indicator when we talk about Mars departure trajectories.
DV TLI is the velocity added during the trans-Lunar Injection burn and DV LOI is the velocity added during the Lunar Orbit Insertion burn.

This table assumes insertion is into a 100 km circular lunar orbit. Note, that these are the resultant velocities and don’t include gravity losses during the burns (more about that later). The shape of these trajectories in the frame rotating with the moon is shown in Figure 5 below. For our purposes we are interested in direct trajectories that take between three and six days to reach the moon. This range of trajectories tend to result in minimum DVs and hence lowest mass in LEO. They were designed to access only low Lunar latitudes, but a small mid course correction will allow insertion into lunar polar orbit instead
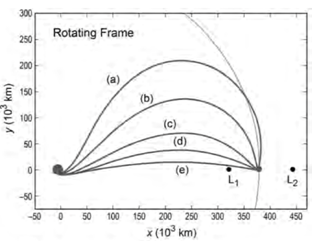
Lunar Orbital Planform – Gateway
NASA is taking an entirely different approach. NASA is base lining the Lunar Orbital Platform – Gateway (LOP-G) which is mini-space station/support facility in a highly elliptical orbit around the moon (periapsis is 3000 km and apoapsis is 70,000 km). NASA intends this facility to be an international facility for planetary science, astrophysics, Earth observations, heliophysics, fundamental space biology, and human health. Fourteen space agencies including NASA have signed up to support this concept. The LOP-G is to be put in a seven-day near-rectilinear halo orbit (NRHO) as shown in Figure 6 below (rectilinear in this case means normal to the Earth-Moon axis).This figure shows the family of all possible L2 halo orbits with the proposed NRHO shown in white. The DVs to reach the LOP-G from LEO are the same TLI burn (3140 m/sec), a shortened LOI burn (180 m/s), and then a 240 m/s burn to rendezvous with the LOP-G at apoapsis. The NRHO appears to require less DV to rendezvous and leave than the previous candidate Gateway orbit which was a halo orbit around L1 (1st Lagrange point between the Earth and the Moon).
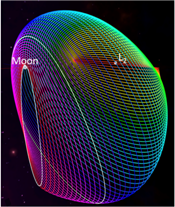
Total travel time from LEO to LOP-G would nominally be approximately 5 days, but there are obvious scheduling issues involved. What if the twice a month TLI launch window doesn’t match up with the once every seven-day NRHO apoapsis? Obviously the LOP-G is going to have to have the capability the change its orbit period to make for timely rendezvous for payloads from Earth, or for unscheduled emergency returns of crewed space craft during landing or from early lunar bases. These were not issues for the earlier Gateway designs because it was in a close halo orbit around the L1 Lagrange point where it had constant availability but required increased DV budgets for access from Earth and LLO. I see this as a serious design issue that really hasn’t been discussed.
I will try and give some background on the LOP-G to explain how we got into this interesting situation. NASA is funded by Congress and gets its nominal agenda from the White House. Unfortunately, when the White House and Congress don’t see eye-to-eye, which has been the norm for the 21st century, NASA tends to flounder with conflicting directions and insufficient funding. This is how we got the Senate Launch System (SLS) and the Orion Crew Transportation System. They were make work projects for NASA Marshall Space Flight Center (MSFC) and NASA Johnson Space Center (JSC) respectively to keep our spaceflight design and development capability alive and well until a new administration with a major commitment to space came along. I don’t agree with the elements they chose to develop (I would have spent the money to develop reusable launch and upper stage systems), but their logic of keeping our space development infrastructure alive was dead on.
Unfortunately, Orion and the SLS took virtually all of the NASA Space Exploration funding available and since there was no succinct directions from the Obama administration, NASA picked what they believed would be a low impact, low cost, next step, which was the Gateway Station (see Figure 7 below) as the follow-on for Orion and SLS development.
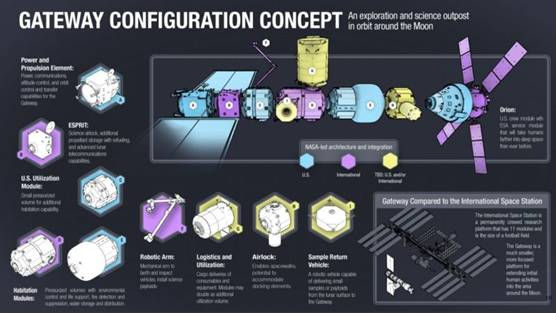
This highly modular station could be launched in sections to the vicinity of the moon by the SLS and enlarged and serviced using the Orion/SLS. It could also be launched in smaller sections using existing launch vehicles from the various countries making up the operating consortium. As you can see it has something for everyone willing to participate and develop space components, so it makes an almost ideal system for an international consortium. It is essentially a small version of the ISS operated above the protection afforded by the Earth’s magnetic field. A science station above the Earth’s magnetic field makes good sense but it isn’t necessary for it to be in the vicinity of the moon and play a vital role in lunar transportation. In fact, the moon complicates access and increases its operating costs. When NASA designed the lunar Gateway station their overall plan was to develop technologies needed for a long-term effort to go to Mars and at the same time support an international effort to further explore the lunar polar regions where water ice should be locked up in crater bottoms. Putting major permanent bases on the moon as Trump has espoused was not in the picture, and hence the SLS/Orion/Gateway system is seriously undersized and an unnecessary obstacle for putting astronauts and bases on the moon.
The concept of operations (CONOPS) for the LOP-G is to assemble and fuel the lunar lander plus its payloads at the LOP-G using multiple flights of the Space Launch System (SLS) and its Centaur upper Stage. The currently funded Block 1 SLS will deliver approximately 26 mT to TLI and the current Falcon Heavy will deliver 22 mT to TLI. This lack of mass to TLI seriously limits any lunar lander concepts. and the two currently proposed concepts are a two-stage system by Lockheed Martin massing 22 mT at TLI and NASA’s Advanced Exploration Lander (AEL) with three stages each massing 10 to 15 mT. Each stage of the AEL would be launched separately to the LOP-G and assembled together for the trip to the surface. The three components of the AEL are; the transfer stage (delivers lander to and from LOP-G from TLI), lander descent stage, and lander ascent stage.
As it happens, NASA is thoroughly locked into LOP-G now.This is because the existing Orion crew transportation system has a total DV capability of 1338 m/s, while an Apollo-like trip from TLI in and out of LLO to transfer crew to the lander takes a DV of roughly 1650 m/s. Therefore, Orion is unable to perform crew transfers to the Lunar Lander unless it harnessed itself to the LOP-G. With the LOP-G in lunar orbit, the Orion only needs 420 m/s to enter lunar orbit and rendezvous, and same 420 m/s to depart the LOP-G and boost into the transfer orbit back to Earth. This provides NASA with additional Orion payload capability for future mission growth.
However, with the lunar lander based at the LOP-G, the lander trip from the LOP-G to polar LLO would require a DV of 730 m/s and half a day. This significantly impacts the lander design since its landing total DV has been increased from 2135 m/s to 2865 m/s, and the ascent total DV from 1860 m/s to 2590 m/s. Based on my old NASA lunar lander design models, if we sized the lunar transportation system to deliver 15 mT to the lunar surface, the total lander mass at departure from the LOP-G would be 60.4 mT and the total lander mass if it was fueled in LLO would be 39.8 mT. Obviously, we’re using a LEO Depot and a new reusable upper stage(and not the SLS) for this part of the exercise. With this new reusable upper stage to deliver payload and propellants from LEO to a transfer station near the moon, and a reusable lunar lander that is refueled at that same transfer point, the total mass that has to be delivered to LEO to deliver the 15 mT to the surface, is199.3 mT if we’re using the LOP-G to transfer propellants and payload, and 103.35 mT if we use LLO as the transfer point. At a launch cost of $1000/kg (typical in the near future if we are using a RLV) we would be paying an extra $96M for each 15 mT delivery using the LOP-G. The bottom line is that the LOP-G made sense for small lunar exploration and science missions but makes no sense for a major lunar base-building operation, and the economics suck.
Transfer Propellants and Payloads in LLO –
Basing the transfer of payloads and propellants in LLO was first proposed during the NASA Space Exploration Initiative (SEI) back in 1989. Figures 8 below show the 1989 Lunar Lander and Orbital transfer vehicles transferring payloads and propellants in LLO. The payloads are in two cargo containers and the transfer propellants are in the two cylindrical tanks next to the upper stage central propellant tanks. The mission shown is a crew return mission. The crew cabin shown on top of the lander will dock with the Upper Stage core, the crew will oversee the transfer of the payload containers and liquid propellants to the lander, and then undock from the lander, which will return to the lunar base with the cargo. The reusable Aerobraking Orbit Transfer Vehicle (ABOTV) will perform the Trans-Earth Injection (TEI) burn and return the Crew Cabin and crew back to the LEO depot. The crew and crew cab get to the moon as additional cargo on the ABOTV.
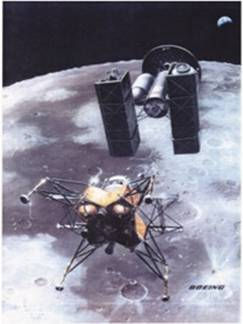
Figure 9 below shows two ABOTVs using the Earth’s upper atmosphere to slow down in order to rendezvous with the LEO depot. This painting shows an updated version of the ABOTV where the propellant tanks have been resized and redistributed to keep the tanks and the crew cab (not shown) out of the super heated air stream.
Using the ABOTV as our upper stage saves most of the 3100 m/s propulsive DV required for the Earth Injection Burn. The Aerobrake structure and TPS mass about 15% of the return weight while the propulsive mass to accomplish the 3100 m/s burn is near 100% of the return mass so the mass savings is significant.
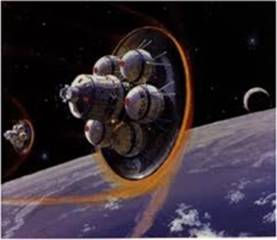
Lunar Direct –
How does the lunar direct scenario compare with LLO transfer scenario? For that I go back to my lunar lander design models. Dr. Zubrin’s plan is to build an oversize lander that does the TLI burn (3150 m/s), the LOI burn (915 m/s), and the landing burn (2135 m/s) with no help from an additional upper stage. That is physically possible, but it does cause some problems. One the lander stage is huge; for 15 mT payload, it is 6 m in diameter, 18 m high (w/o payload), and masses 145.5 mT in LEO, but that is still within the capability of the Block 2 SLS and could launched with two flights of the Space-X Falcon Heavy. The other problem is refueling on the lunar surface to return home. The lunar direct lander with 15 mT payload capability holds 111.25 mT of LOX/LH2 propellant which is about six times the size of the propellant loads I’ve studied in the previous lunar landers studies I’ve participated in. In previous studies outposts were followed by mines and production facilities which produced hydrogen and oxygen as byproducts (main products were iron, nickel, and Platinum Group Metals). The hydrogen and oxygen byproducts could supply 20 mT of propellants for a launch every other month, but it would take a year to amass enough propellants for the Lunar Direct Lander and 15 mT of payload delivered per year would not support a lunar mining complex.
Therefore, the Lunar Direct approach is going to have to land at the lunar base at the poles where we believe there is abundant water frozen in the regolith. Unfortunately, the poles do not seem to be rich in nickel/iron (Platinum Group Metals) or Thorium (Rare Earth Metals) so it makes little sense to build mining outposts there. It is possible to refuel the Lunar Direct Lander at the poles and “hop” to a base or mining site as far as the equator. To transfer 15 mT of payload to a site on the equator requires the entire 111.35 mT of LOX/LH2 propellant (two ascents and two landings). There are two issues with this approach. Lunar landings are not that easy or safe, as shown by the recent failures of both the Israeli and Indian lunar landers (2019), so we have always book kept a failure rate of 1% for lunar landings. This means a Lunar Direct mission to deliver a payload to an outpost or mining camp away from the poles has three times, or 3% probability of vehicle loss before returning to Earth. That is a significant increase in loss of mission probability and will impact operating costs.
The second issue is the cost of propellant. Propellant is not free at the lunar poles. The regolith permafrost needs to be mined and concentrated, then heated in a suitable container to separate out the various gases, which then will be distilled to separate pure water from the other gases (like CO2, N2, ammonia, etc.).The pure water will then be electrolyzed into gaseous H2 and O2, which needs to be liquefied and stored as cryogenic liquids. The lunar propellants plant in previous studies could be delivered by single lander but now we're talking about something an order of magnitude bigger.
If we stay with our traffic model for lunar mining, we are averaging six 15 mT payloads per year to the lunar surface per mining site. Assuming the mines are not at the lunar poles that level of traffic is going to require approximately 1000 to 1335 mT of LOX/LH2 propellant per year per outpost or mining site. This is equivalent to a small $3B liquefaction plant on Earth, but it’s not on Earth. There are some advantages for a liquefaction plant at the lunar poles. One is the ability to radiate heat directly to deep space (~ 2.7 deg K), but most of the power to liquefy hydrogen (~ 10 kWh/kg) is used for compression and the colder heat sink will not significantly impact that. The bottom line is that with Lunar Direct each outpost or mining site will require roughly $20B of mining, processing, and liquefaction facilities and 100 kWe of steady power at a lunar pole. This won’t come cheap.
There has been much discussion of mining water at the lunar poles for propellants to go to Mars, but if you run the economics and the orbital mechanics, LOX/LH2 is more valuable in LEO (and probably cheaper) than it is in a lunar halo orbit if your goal is to get to Mars. This is because of the Oberth effect where thrust applied deep in a gravity well multiplies the exit velocity, so less propellant is required to get an expedition mass to the required Mars C3 if most of the burn is done in orbital passes near Earth.
I modified a spreadsheet I used on previous NASA studies to compare the three options discussed here. That spreadsheet is shown as Figure 10 below. This table shows the payloads, the inert masses and the propellant masses for each of the three options with payload masses of 15, 30, and 50 mT. To make the comparison equal in all three cases I have assumed that all lander propellants are produced on the moon. That is a requirement for Lunar Direct, but it also benefits the LOP-G and LLO Transfer concepts.
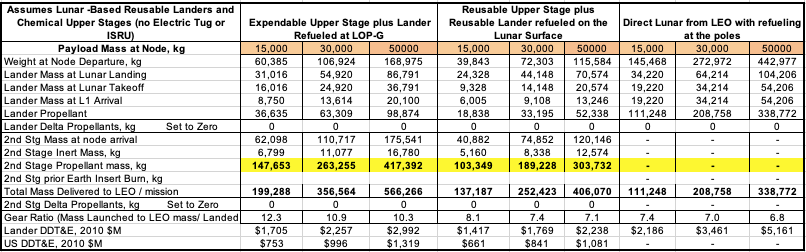
Notice the term Gear Ratio is in the table. Gear Ratio is how we measured the efficiency of lunar payload delivery back in 1989. Gear Ratio is the mass launched to LEO divided by the delivered payload mass. The Apollo program had a gear ratio near 22 while the LOP-G has a Gear Ratio near 12 and the LLO Transfer has a Gear Ratio near 8. Lunar Direct has a gear ratio near 7.5 which is even better. It also shows the estimated Design, Development, Test & Engineering (DDT&E) costs for each vehicle. Development cost wise, all the transportation vehicles required are about comparable. Note, that the $6.6 B cost for the LOP-G is not included in these numbers. If we were to book keep the total transportation systems costs for each of these three systems sized to service two outposts and three mining camps, I would estimate $28B for LOP-G (5 landers, 3 ABOTVs, five 220 mT/yr propellant plants and 1 LOP-G); $15B for LLO transfer (5 landers, 3 ABOTVs, and five 113 mT/yr Propellant plants); and $60 B for Lunar Direct transfer (5 landers, no ABOTVs, and a single 6000 mT/yr propellant plant). The bottom line for lunar delivery is that all three options presented here will physically work, but LOP-G will significantly increase costs relative to the LLO transfer (about double if you include the costs of the LOP-G itself), while the Moon Direct will increase costs by a factor of four relative to LLO Transfer because of the amount of propellant processing infrastructure required on the moon. Therefore, I recommend we focus on LLO Transfer for any Return to the Moon program.
Comments